From Chemistry to Electricity: How Batteries Work
Unlocking the energy vault: the what, how and why of batteries
Welcome to our third episode on Distributed Energy Resources (DERs) 👋🏼
Today, we’ll focus on batteries. Namely electrochemical batteries. Without further ado, a round of 👏🏼 for the 🔋!
What Is a Battery?
A battery is a storage container for chemical energy. Upon request, the chemical energy converts into electricity. The resulting electricity can power anything that relies on electricity. We cannot store electricity directly, hence the workaround through chemical energy.
🧪 + 🪨 = ⚡️ —> 🔋
The chemical energy is stored in metals such as lithium (Li), sodium (Na), or nickel (Ni). The first batteries contained combinations of lead-acid, then came nickel-zinc, then sodium-sulphur, and eventually lithium-ion entered the arena.
Today, the most common batteries are lithium-ion. Ions are atoms with a net electrical charge either positive or negative. There are different types of batteries but almost all of them have lithium as a core component.
Every battery has a few essential parts:
Anode: The negative end of a battery. Gives excess electrons. Made from a material mix such as lithium-cobalt-oxide or lithium-iron-phosphate.
Cathode: The positive end of a battery. Receives excess electrons. Made from a carbon-based material such as graphite or silicon.
Electrolyte solution: A chemical solution that enables the flow of electrical charge between anode and cathode. For example, it transports lithium ions in a lithium-ion battery.
Separator: Allows flow of ions between cathode and anode but prevents electrons from flowing on the same route. Ergo forces them to go through an external circuit.
Negative current collector: Receives electrons when the battery is charging.
Positive current collector: Receives electrons when the battery is discharging.
Energy Density of a Battery
The choice of materials is important. Each combination has its pros and cons. Some materials are better at holding energy than others. But these materials might be more expensive. One key aspect of a battery’s quality is its energy density.
Energy density describes the energy contained in the battery proportionally to its weight. The metric used to measure this is Watt-hours per kilogram (Wh/kg). Remember we learned about kilowatt hours on your annual utility bill? This is the same. One Watt-hour describes the consumption of one Watt for one hour.
With batteries, we’re trying to find a sweet spot between energy density, cost, and size. It’s not ideal to have a high energy density when it’s not commercially viable. The same applies to extremely cheap batteries if they don’t have a solid energy density. You get the dynamics that we’re trying to optimize for.
We have different batteries for different purposes. A phone battery has a different use case than an EV. But in principle, they all work the same.
How Does a Battery Work?
Batteries create electricity by converting the chemical potential (stored energy) into electricity. When an electric circuit completes, chemical reactions occur on either side of the battery. The chemical reactions between the two metals and the electrolyte allow the electrons to flow from one side to the other, thus creating electricity.
Let’s look at a Lithium-ion battery in action. The Department of Energy created a great visualization for the flow of electrons using an EV as an example:
Lithium ions travel through the electrolyte and separator
Electrons flow through an external circuit powering the electric car
Charging the battery reverses this process
Think of it this way:
The chemical energy we store inside a battery is little balls of energy. By charging the battery, we move those energy balls up a mountain. When we demand power, those little energy balls roll down the mountain. Through the balls rolling down, energy from their movement can be harnessed to power a device. Once all the balls rolled down the mountain, there is no more energy left. We need to move the balls back up the mountain to be able to repeat the cycle. Time to recharge.
When charging a battery, the flow of electrons reverses through the external source of energy. Every time you recharge a battery, it loses a small amount of its potential. After 500-1000 recharges, the battery lost significant potential and should be replaced.
Why Do We Need Batteries?
We need them because they allow us to move energy through time. We create energy now and use it later. Isn’t that cool?!
Batteries transformed many sectors in the past and will continue to do so going forward. They have a giant disruptive potential. Soon they will conquer shipping, long-haul trucking, and eventually short-distance aviation.
Traditionally, we have a consumption-following electricity generation. We generate electricty based on consumption forecasts. With batteries, we can shift that paradigm to consumption-forming generation. When favorable, we to store excess electricity in the form of chemical energy. When needed, we convert that chemical energy into back into electrictity.
As we start to electrify as many devices and processes as possible, it is crucial to have a flexible and reliable electricity supply. Batteries are here to provide exactly that. On top of that, by combining battery capacities with a Virtual Power Plant (VPP), we can automate and optimize the charging time to when it’s most opportune. Thank you, technology and software.
I’d like to highlight that we already have millions of batteries driving around. EVs potential to serve as a backup solution for when things get tough is often overlooked. All the batteries powering EVs can play a tremendous role in providing grid flexibility.
Where Do Batteries Come From?
Batteries are made from raw materials such as lithium, nickel, or cobalt. These materials have to be sourced, refined, and eventually made into a battery.
Let’s look at the value chain process for batteries:
Mine raw materials from Earth
Process materials into essential components such as sulfate or carbonate
Produce battery cell components such as cathode
Manufacture battery cells
Assemble battery into final product by original equipment manufacturer (OEM) such as Tesla in cars or Apple in iPhones
The end-consumer purchases the final product
Recycle end-of-life batteries
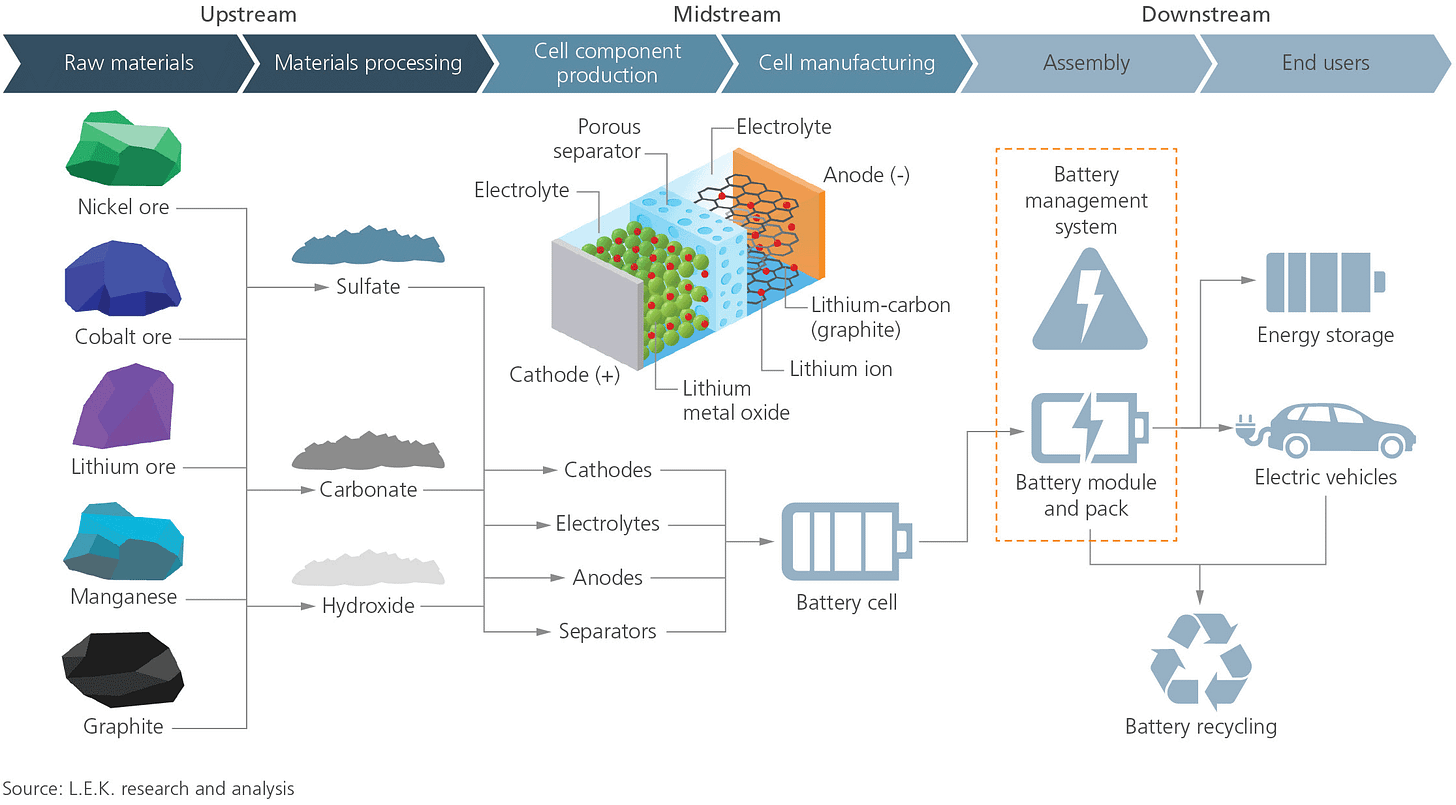
Before this cycle can kick off, we need to find those deposits of raw materials somewhere on the globe. As it turns out, a vast majority of those are geographically concentrated. The so-called Lithium Triangle between Chile, Argentina, and Bolivia is home to the largest lithium reserves in the world. The Democratic Republic of the Congo is the largest source of cobalt.
There are already intense trade wars going on over access to those resources. Securing a reliable supply chain will be essential for future economic success.
When moving on in the value chain, we meet an old friend again - China.
66% of the world’s lithium processing takes place in China. 77% of the global battery supply is manufactured in China. China is leading in battery supply, demand, and innovation:
Dominating the supply chain in almost every phase
Leading global battery demand but the others are catching up
Filing the most patents every year to protect their cutting-edge battery technology.
China realized the potential of batteries early on. Between 2016 and 2022, they spent $57 billion to incentivize EV purchases domestically. That’s 5 times more than the US over the same period. By 2023, the market worked independently and didn’t need government intervention anymore to stimulate it.
The US and Europe are trying to minimize their dependency on China by ramping up supply chain capacities locally. Through legislative pieces such as the Inflation Reduction Act (IRA) and the EU Green Deal, they want to incentivize companies to build out manufacturing capacities on their respective continents.
Where Do We Go from Here?
🤯 Batteries have the potential to help reduce 22 gigatons of CO2. That’s 60% of global energy-related emissions.
Batteries are forecasted to go through the same growth period solar did a decade ago. The analogy fits because batteries also follow learning curves. It’s a reinforcing loop in which more deployment results in cheaper and better batteries which increases demand. With every doubling of battery deployment, cost falls 19-29%, and energy density rises 7-18%
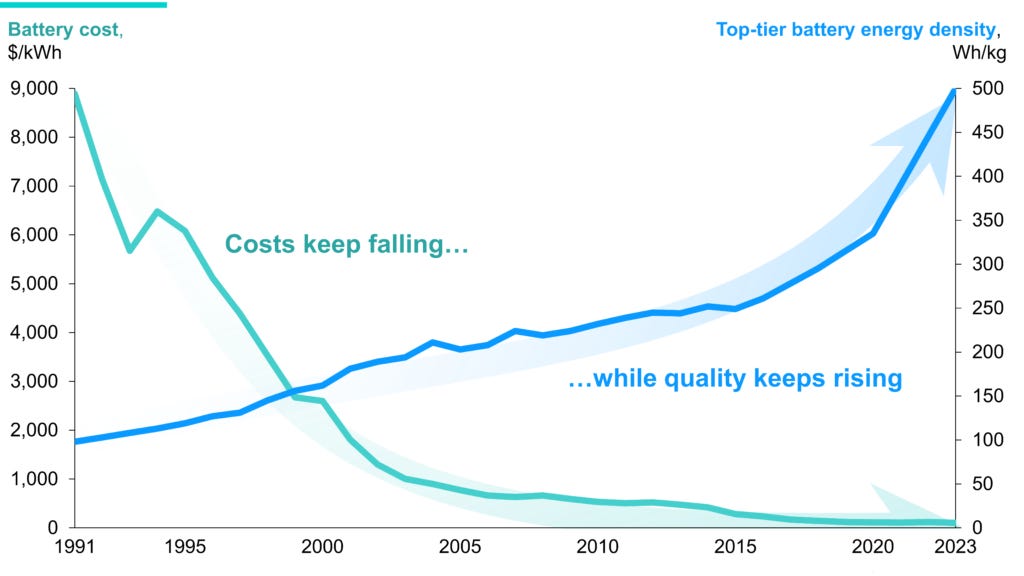
The RMI, a non-profit research institute focused on global energy systems, referred to battery’s progress as the domino effect. It spreads from sector to sector and from region to region. It started with consumer electronics and has taken the mobility sector by storm. More than 20% of global car sales are EVs now.
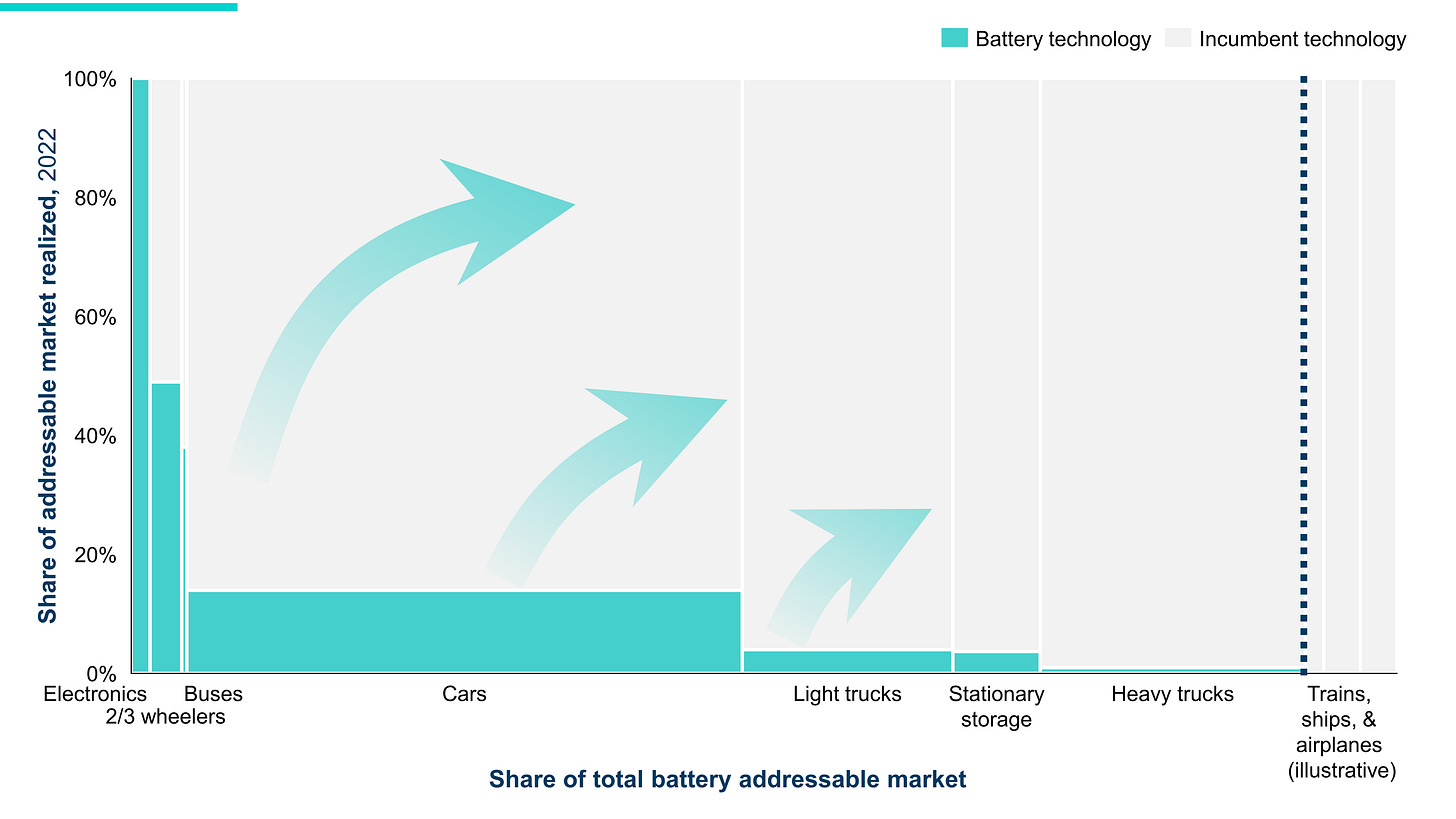
With energy density rising, other sectors will fall as well because it becomes economically viable. Ultimately, the best incentive to switch to electricity powered-engines, heaters, and coolers is by making them price competitive.
In 2022, humankind invested more money in battery factories ($45B ) than in solar and wind capacities combined. The infrastructure is built to supply the growing demand. The fierce competition between China and the rest of the world will only accelerate innovation. This will benefit the market - more competition leads to lower prices and better quality. We are facing exciting times ahead. Batteries have been perpetually underestimated. Forecasts always missed the speed of battery innovation and deployment.
Let’s watch the battery takeoff live and in color from here onwards!
Before I let you go this week, I wanted to share some incredible resources I came across while writing this piece:
Ben James - The Big Guide to Batteries
RMI - Report on domino effect
Inside a battery factory of CATL in China
If you learned something today, please comment below your favorite takeaway. If you didn’t learn anything, let me know what I could have done better. The next time you charge a battery, think of all the little energy balls you are moving through time!
Stay electric,
Basti